Comparative Life Cycle Assessment Of Mycelium Insulation And Expanded Polystyrene Insulation
2024
Carnegie Mellon University
w/ Bright Aungsuthar, Nandan Bhusry, Mariam Vashakidze, Dalia Zainal
Carnegie Mellon University
w/ Bright Aungsuthar, Nandan Bhusry, Mariam Vashakidze, Dalia Zainal
This study compares the insulation properties of mycelium-based insulation to conventional Expanded Polystyrene Foam (EPS) using a cradle-to-gate Life Cycle Assessment (LCA). Focusing on raw material extraction and manufacturing, the study aims to demonstrate how integrating mycelium insulation during construction can improve efficiency and reduce environmental impact. It addresses the gap in data regarding mycelium’s insulation properties and targets those interested in minimizing the environmental impact of building materials. The findings will be shared with the academic community at Carnegie Mellon University engaged in environmental LCA studies.
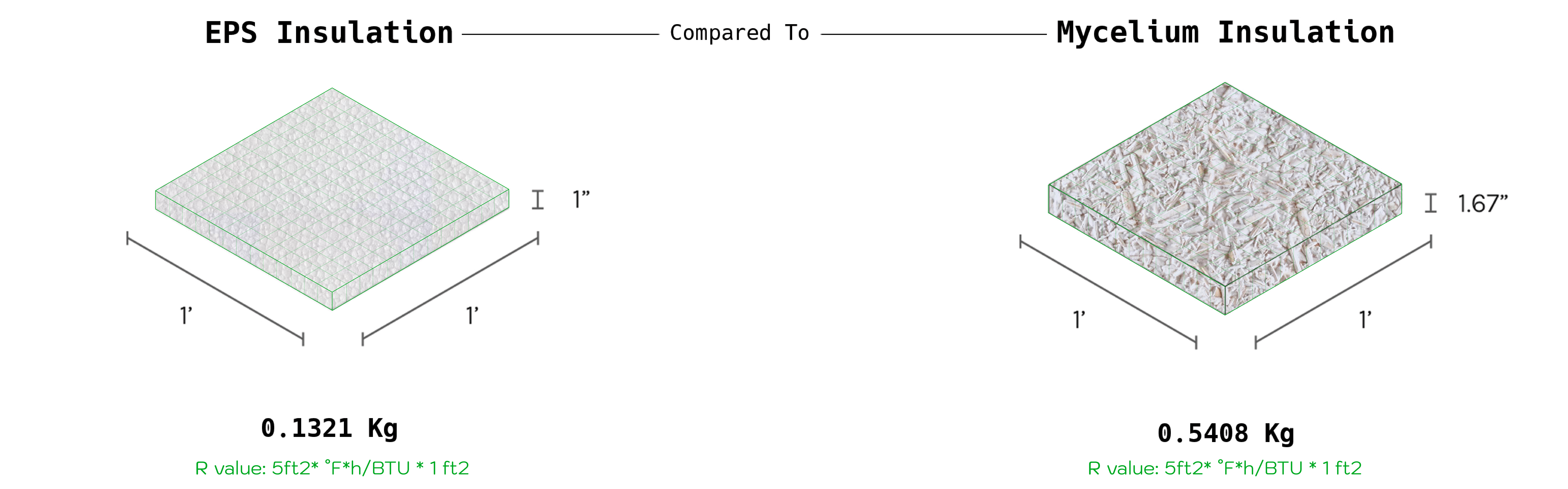
To incentivize scaling up of mycelium insulation and determine the potential environmental hotspots of the production of mycelium insulation.
AUDIENCE:
EPS insulation manufacturers, architects, engineers.
FUNCTION/ UNIT:
To provide thermal insulation in building envelope walls in buildings by withstanding thermal conductance.
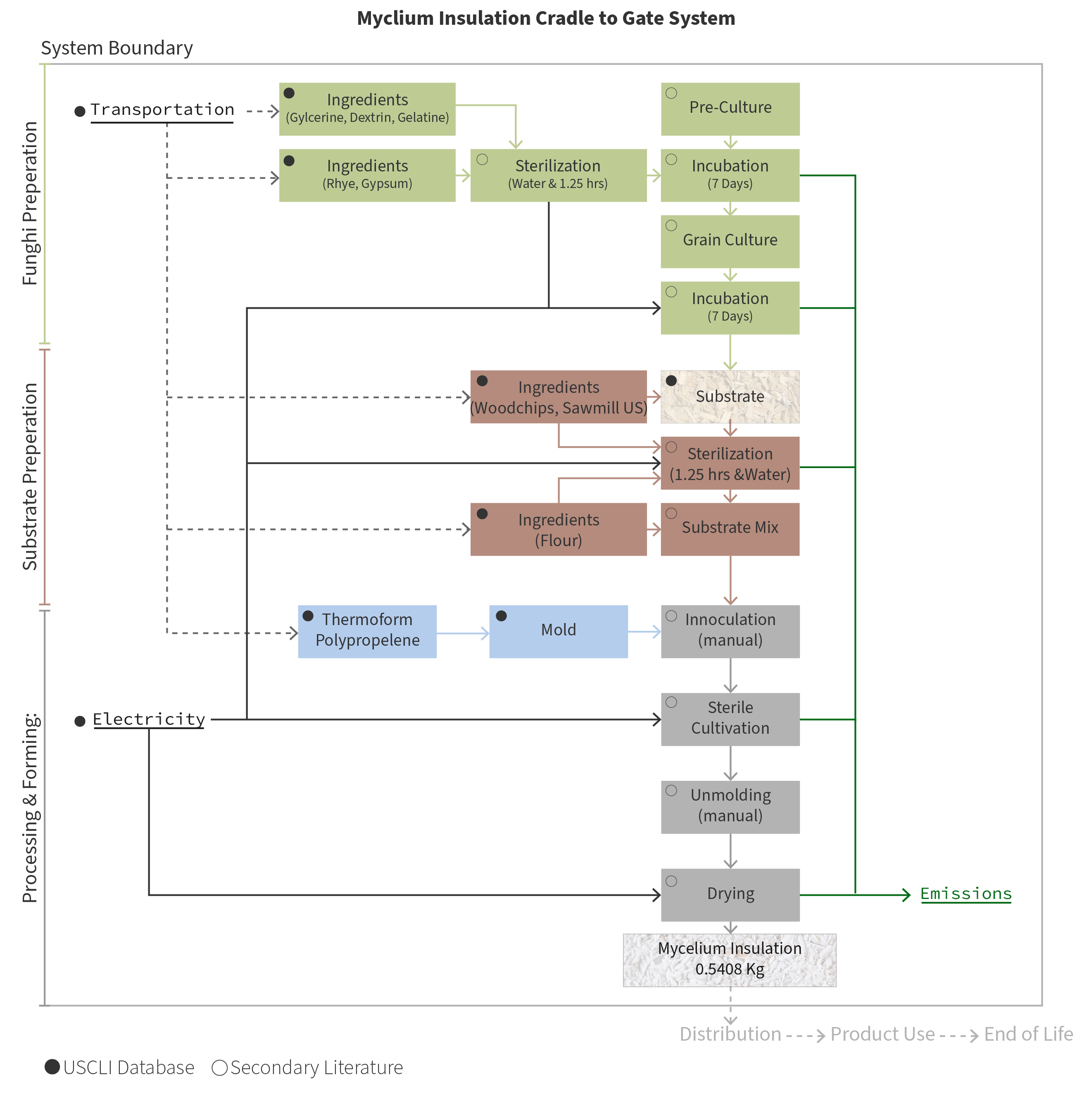
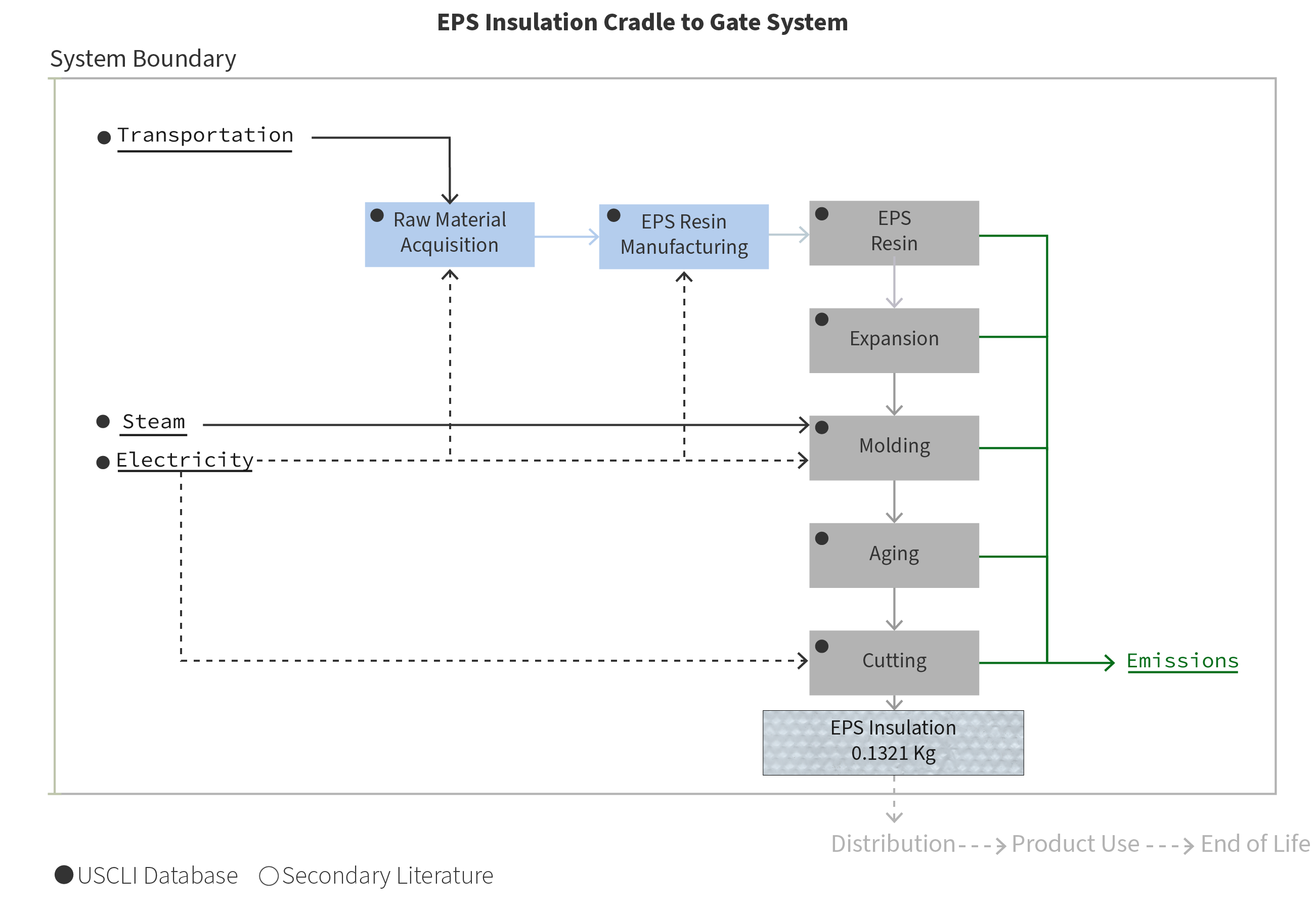
The life cycle assessment (LCA) of mycelium-based insulation was conducted using the OpenLCA software framework, with environmental impacts quantified through the TRACI 2.1 methodology. Primary data was sourced from studies by Steltzer et al., 2021, and Xing et al., 2018, ensuring a reliable foundation. The mycelium insulation process utilized glycerin, dextrin, gelatin, and rye, processed through energy-intensive sterilization and incubation. Inputs were allocated per functional unit, with transportation modeled based on typical North American logistics. Similarly, the cradle-to-gate LCA for expanded polystyrene (EPS) insulation involved data from Franklin Associates and the US Life Cycle Inventory Database. Raw materials such as styrene, petroleum, and natural gas were used to produce EPS resin, which was pre-expanded, molded, and cut into boards. The analysis included transportation impacts and accounted for material disposal from cutting, indicating a negligible effect on overall impact. Both assessments provide insight into the environmental footprint of mycelium and EPS insulation from raw material acquisition through production.
Inventory Output results:
![]()
The inventory input results are reflected in the output, showing that the high energy consumption of mycelium on the grid corresponds to its elevated emissions. The study presents total emissions for each alternative, highlighting the highest environmental and health impacts. Carbon dioxide emerges as the most significant emission, with mycelium powered by the grid contributing the most. However, mycelium with hydroelectric power demonstrates that emissions can be significantly reduced by transitioning to renewable electricity sources. By substantially lowering emissions, mycelium insulation becomes competitive with EPS insulation.
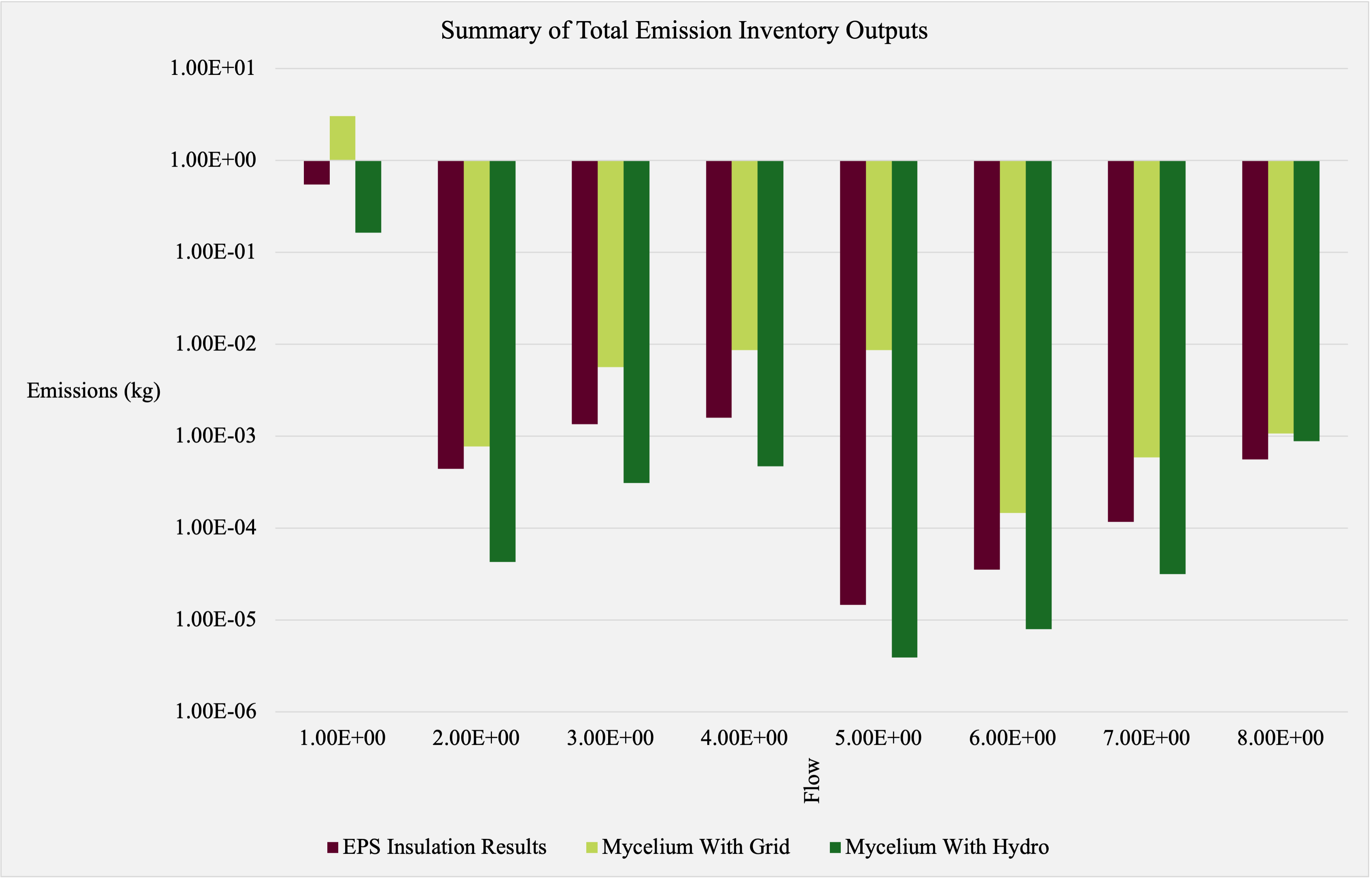
The inventory input results are reflected in the output, showing that the high energy consumption of mycelium on the grid corresponds to its elevated emissions. The study presents total emissions for each alternative, highlighting the highest environmental and health impacts. Carbon dioxide emerges as the most significant emission, with mycelium powered by the grid contributing the most. However, mycelium with hydroelectric power demonstrates that emissions can be significantly reduced by transitioning to renewable electricity sources. By substantially lowering emissions, mycelium insulation becomes competitive with EPS insulation.
Impact Assessment:
![]()
Mycelium insulation powered by grid electricity showed the highest environmental impacts across all TRACI categories. Given the energy-intensive nature of its manufacturing process, switching to hydroelectric power significantly reduced these impacts. The assessment, conducted using OpenLCA, aimed to better understand the environmental implications of the inventory outputs. While all TRACI impact categories were evaluated, the focus was primarily on global warming potential (GWP), as highlighted by existing literature. Modeling the scenario with hydroelectric energy demonstrated a notable improvement in the environmental performance of mycelium insulation.
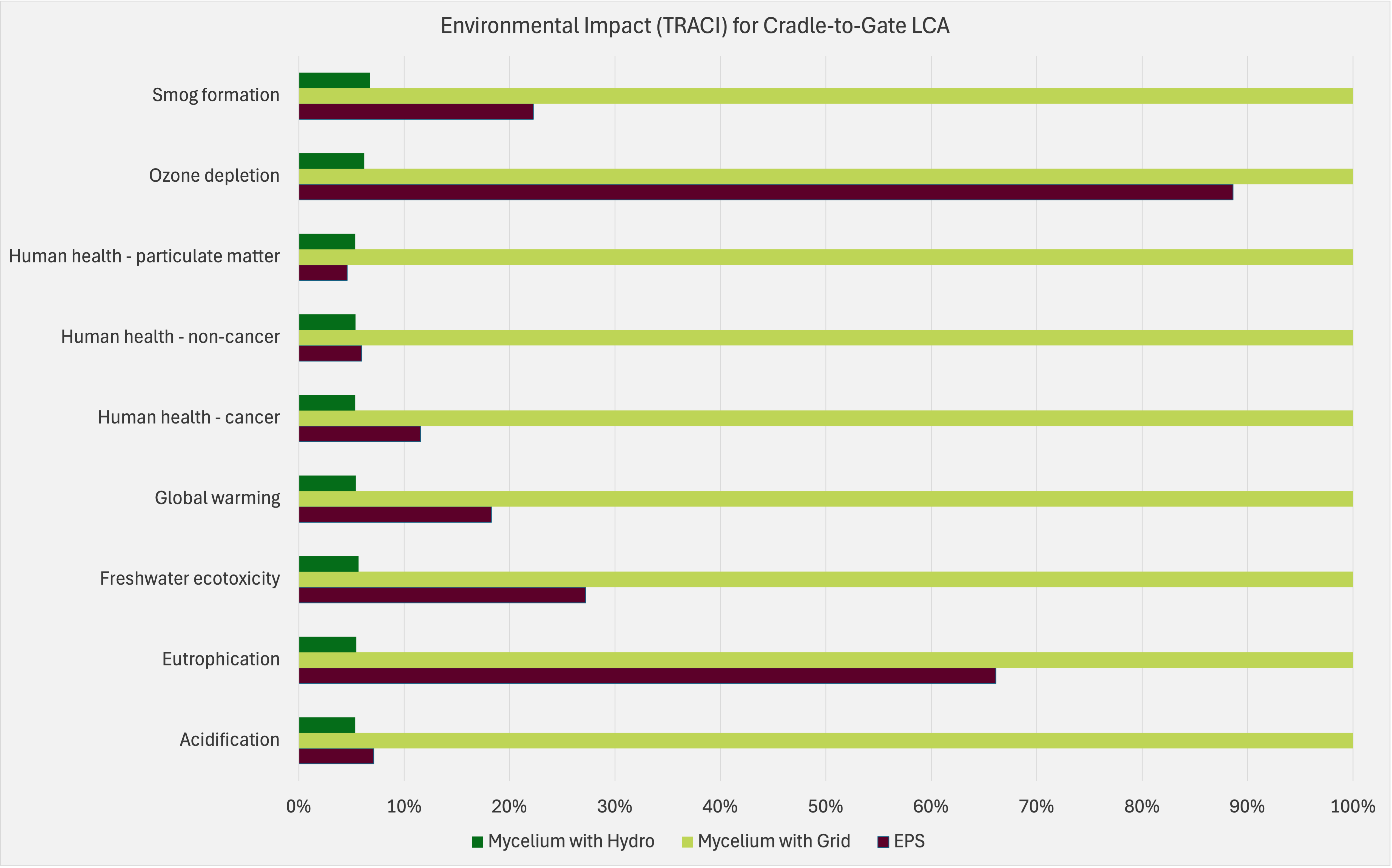
Mycelium insulation powered by grid electricity showed the highest environmental impacts across all TRACI categories. Given the energy-intensive nature of its manufacturing process, switching to hydroelectric power significantly reduced these impacts. The assessment, conducted using OpenLCA, aimed to better understand the environmental implications of the inventory outputs. While all TRACI impact categories were evaluated, the focus was primarily on global warming potential (GWP), as highlighted by existing literature. Modeling the scenario with hydroelectric energy demonstrated a notable improvement in the environmental performance of mycelium insulation.
Global Warming Potential:
![]()
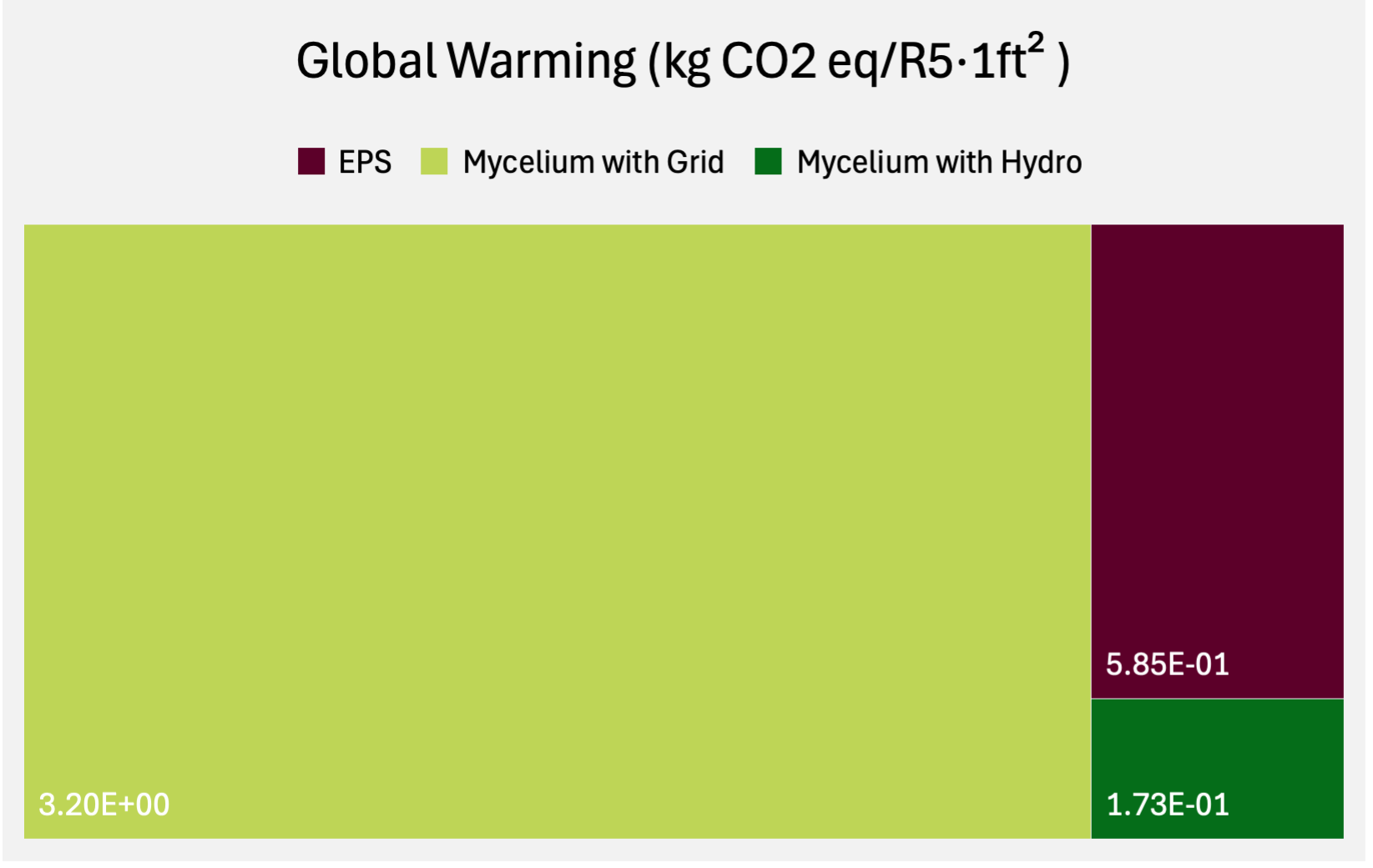
Hot Spot Analysis:
![]()
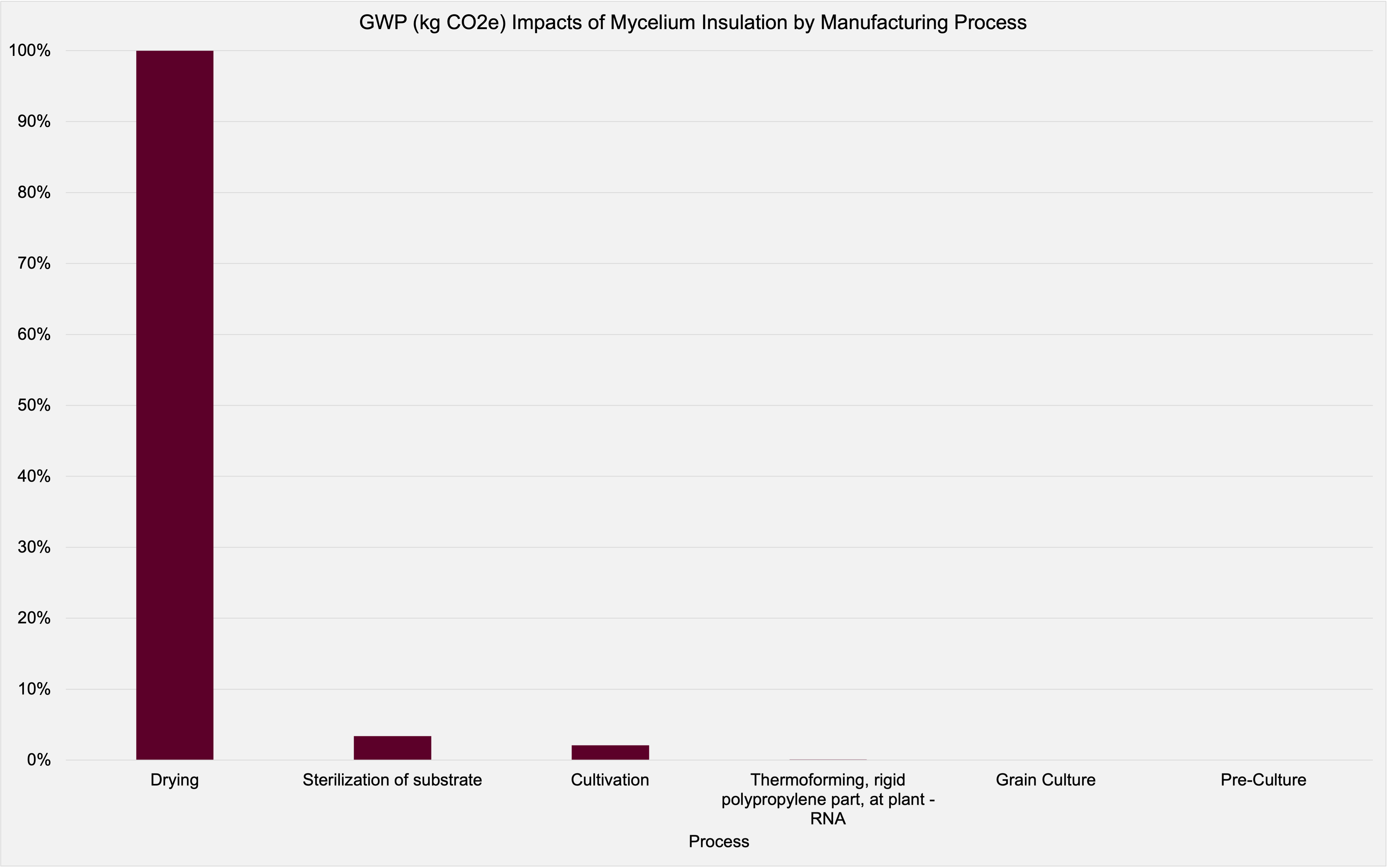
The drying process of mycelium using grid electricity accounted for 94.6% of its global warming potential (GWP), making it the most significant contributor. This process, conducted in an industrial oven, results in a high energy load and associated GWP. Additionally, the drying process was responsible for 93% of the carbon dioxide emissions, underscoring the need to improve the sustainability of mycelium insulation production. To align the GWP of mycelium insulation with that of EPS, the adoption of renewable energy or the development of innovative drying strategies is crucial. Reducing GWP for EPS could also be achieved by optimizing transportation routes and promoting local manufacturing.
Insight:
Due to the lack of specialized equipment for mycelium production, agricultural machinery was used, leading to some uncertainty in the data. Future steps include gathering primary data for industrial-scale applications. Carbon and waste sequestration are not yet factored into the process due to challenges in quantification.
Due to the lack of specialized equipment for mycelium production, agricultural machinery was used, leading to some uncertainty in the data. Future steps include gathering primary data for industrial-scale applications. Carbon and waste sequestration are not yet factored into the process due to challenges in quantification.
References:
NREL. (2023, December 22). “EPS insulation board, at plant - RNA” U.S. Life Cycle Inventory Database. Retrieved from National Renewable Energy Laboratory. https://www.lcacommons.gov/lca-collaboration/National_Renewable_Energy_Laboratory/USLCI_Database_Public/datasets. Accessed 1 April 2024.
Alaux, N., Vašatko, H., Maierhofer, D., Saade, M. R. M., Stavric, M., & Passer, A. (2023). Environmental potential of fungal insulation: a prospective life cycle assessment of mycelium-based composites. The International Journal of Life Cycle Assessment, 1-18.
Stelzer L., Hoberg F., Bach V., Schmidt B., Pfeiffer S., Meyer V., & Finkbeiner M. (2021). Life Cycle Assessment of Fungal-Based Composite Bricks. Sustainability, 13(21), 11573. https://doi.org/10.3390/su132111573
NREL. (2023, December 22). “EPS insulation board, at plant - RNA” U.S. Life Cycle Inventory Database. Retrieved from National Renewable Energy Laboratory. https://www.lcacommons.gov/lca-collaboration/National_Renewable_Energy_Laboratory/USLCI_Database_Public/datasets. Accessed 1 April 2024.
Alaux, N., Vašatko, H., Maierhofer, D., Saade, M. R. M., Stavric, M., & Passer, A. (2023). Environmental potential of fungal insulation: a prospective life cycle assessment of mycelium-based composites. The International Journal of Life Cycle Assessment, 1-18.
Stelzer L., Hoberg F., Bach V., Schmidt B., Pfeiffer S., Meyer V., & Finkbeiner M. (2021). Life Cycle Assessment of Fungal-Based Composite Bricks. Sustainability, 13(21), 11573. https://doi.org/10.3390/su132111573
USDA Forest Service. (2022). Timber Product Output and Use, North Carolina. Asheville: United States Department of Agriculture.
USG. (2024, April 8). Manufacturing Locations. Retrieved from USG: https://www.usg.com/content/usgcom/en/about-usg/worldwide-locations/manufacturing-locations.html. Accessed 30 March 2024.
U.S. Forest Service Forest Product Lab. (2019, Dece,ber 20). “Chips; softwood; dry; at planer” U.S. Life Cycle Inventory Database. Retrieved from U.S. Forest Service Forest Product Lab: https://www.lcacommons.gov/lca-collaboration/US_Forest_Service_Forest_Products_Lab/Woody_biomass/datasets. Accessed March 30.
USG. (2024, April 8). Manufacturing Locations. Retrieved from USG: https://www.usg.com/content/usgcom/en/about-usg/worldwide-locations/manufacturing-locations.html. Accessed 30 March 2024.
U.S. Forest Service Forest Product Lab. (2019, Dece,ber 20). “Chips; softwood; dry; at planer” U.S. Life Cycle Inventory Database. Retrieved from U.S. Forest Service Forest Product Lab: https://www.lcacommons.gov/lca-collaboration/US_Forest_Service_Forest_Products_Lab/Woody_biomass/datasets. Accessed March 30.
Xing, Y., Brewer, M., El-Gharabawy, H., Griffith, G., & Jones, P. (2018, February). Growing and testing mycelium bricks as building insulation materials. In IOP conference series: earth and environmental science (Vol. 121, p. 022032). IOP Publishing.